COMPARATIVE ANALYSIS OF MISCIBLE AND IMMISCIBLE GAS FLOODING IN ENHANCING OIL RECOVERY
CHAPTER ONE
INTRODUCTION
BACKGROUND OF STUDY
Enhanced oil recovery (EOR) is a type of oil recovery which involves the injection of materials not originally present in the reservoir. Enhanced oil recovery processes are targeted at recovering oil which was unrecoverable by conventional approach.
In the past 40 years Carbon dioxide (CO2) flooding has been used as a commercial process for enhanced oil recovery (EOR) and has been reported to be the second most applied EOR process in the world (Jarrell et al., 2002; Di et al., 2011). Field applications as well as laboratory studies have proved that CO2 can be an efficient oil-displacing agent.
It is been considered that the injection of CO2 in mature hydrocarbon fields remains a favorable option to reduce atmospheric CO2 accumulation and thus alleviate the effect of greenhouse on climate (Bradshaw and Cook, 2001; Di et al., 2011; Tian et al., 2015).
CO2 flooding has been field tested for oil recovery with varying degrees of success. Application of CO2 injection in heavy oil reservoirs has received less attention compared to light oil reservoirs. There are two reported reasons for this; it is believed that in heavy oil reservoirs, CO2 lacks acceptable sweep efficiency due to the large viscosity contrast between CO2 and oil as well as unlikely development of a miscible front in heavy oil reservoirs.
The extent of oil recovery is influenced by a number of parameters such as relative permeability, wetting conditions, viscous fingering, gravity tonguing, channeling and the amount of crossflow/mass transfer. Tang et al. observed that the morphology of flowing gas bubbles plays a dominant role on solution gas drive in heavy oils. Relative permeability is one of the essential parameters required in numerical simulators to design and make a decision for any reservoir development.
Singh et al., (2010) compared the viscous and gravity dominated gas-oil relative permeabilities and suggested that gas flood relative permeability can be applicable to viscous dominated regions of the reservoir as long heterogeneities and flow rates are accounted for properly.
Al-Wahaibi et al. investigated the behaviour of two-phase drainage and imbibition relative permeabilities at near miscible conditions and concluded that as the interfacial tension decreases, the non wetting phase relative permeability increases more rapidly than the wetting phase relative permeability.
Schembre et al. examined the effects of temperature on heavy-oil relative permeability and found that diatomite rocks became more water-wet with temperature. Sola et al.] investigated temperature effects on the heavy oil/water relative permabilities of carbonate rocks and observed that the shape of oil relative permeability changes with increasing temperature.
This was attributed to wettability alterations due to elevated temperature. Their results were in contrast to some previous studies dealing with sandstone systems where residual oil saturation was found to decrease and irreducible water saturation increases with temperature.
Dana and Skoczylas show that, for a viscosity ratio the gas relative-permeability remains unchanged even if the wetting fluid viscosity is twenty times higher than that of water. Viscous fingering is a phenomenon that arises due to the instability of the interface between two fluids that have different viscosities.
This takes place either on the front or rear of sample plug when there is a sufficiently high viscosity contrast between the displacing and displaced fluid Viscous fingering and dispersive by-passing is found to increase with oil viscosity. The viscosity of the oil phase has a profound effect on coalescence dynamics of gas during bubble nucleation. Brailovsky et al., suggested that for both immiscible and miscible displacement, instability is caused by the high mobility of the displacing fluid.
This is attributed mainly to viscosity and/or density stratification. Musuuza et al. examined stability criteria for density driven flows in homogeneous porous media. It was suggested that a stable system is attained when there is a balance of external forces such as inertia, viscous stresses and buoyancy.
The effect of asphaltene content on the heavy oil viscosity has long been studied, which shows that increasing asphaltene contents result in an increase of oil viscosity. Peng et al. concluded from their studies on oil chemistry, that acid and base groups within asphaltene are a source of interfacial instability. Viscosity reduction in heavy oil by CO2 has been reported to be much larger than in light oil.
The problem of the asphaltene-deposition-induced formation damage during oil production is increasingly emphasized in the petroleum industry. Asphaltene deposition can occur not only inside the reservoir but also in the wellbore, tubing, and surface facilities, which can plug the porous media, reduce the permeability of the reservoir, and damage the production equipment.
It is especially severe when accompanied by gas injection during oil production. Therefore, gas-injection-induced phase behavior change of asphaltenes is a significant matter requiring research. Experimentally determining the thermodynamic conditions at which asphaltene deposition occurs would be ideal. However, the experiments require a large amount of on-site live oil (crude oil that maintains the original thermodynamic state of the reservoir), which is prohibitively expensive and live oil sometimes cannot be obtained for experiments.
Thus, in order to determine the thermodynamic conditions of asphaltene deposition more intuitively and conveniently, a prediction model can be established according to the obtained experimental data and the law of thermodynamic changes.
Various model approaches have been suggested in the literature for predicting asphaltene deposition such as solubility models, solid models, colloidal models, and associations of the equation of state (EOS) models. Solid models proposed by Nghiem et al. were completely based on the EOS and the gas-liquid-solid equilibrium calculation method was used to determine the change in fugacity caused by temperature, pressure, and volume changes.
Therefore, the phase state of asphaltenes can be determined by comparing the fugacity coefficients of asphaltenes in three phases and, ultimately, the thermodynamic conditions of asphaltene deposition can be determined. Through analysis of the existing prediction model of asphaltene deposition, solid models were selected in this paper. The asphaltenes were considered to be a solid phase, and the asphaltene deposition problem was treated by liquid-solid equilibrium.
When the temperature, pressure, and composition of the crude oil change, asphaltene deposition occurs due to the broken phase equilibrium of the system. In order to determine the asphaltene deposition conditions, the complex multi-phase equilibrium of the system is involved. It was necessary to introduce the EOS for phase equilibrium calculation.
For the gas, liquid, and solid three-phase equilibrium of the system, the cubic EOS is better especially when represented by the Soave-Redlich-Kwong (SRK) and Peng-Robinson (PR) equations. Past studies have proven that the PR equation is particularly suitable for the asphaltene flocculation and deposition calculation [22–24]. Since the composition of crude oil is complicated, obtaining its detailed chemical composition is difficult.
Therefore, the critical parameters in the EOS are generally treated with some special mathematical methods called the characterization method, which includes the estimation of critical parameters (critical temperature, critical pressure, and critical volume), calculation of the interaction coefficient, and division of pseudo-components.
STATEMENT OF THE PROBLEM
It is a known fact that the term “easy oil” refers to the oil that can be extracted easily in inhabitant areas is now vanishing and the amount of oil produced by the primary recovery from these areas accounts only 20 to 30 % of the total amount available.
[2] Petroleum companies are looking for oil in a very remote areas; like deep waters, areas where the temperature is below zero and to develop fields in areas like these is very costly, instead we can still produce the remaining amount of oil in the existing fields by applying new technologies to increase the recovery factor through introducing the enhanced oil recovery techniques.
By applying the enhanced oil recovery techniques millions of barrels of oil can be extracted from existing fields, as it increases the recovery up to 60 % of the oil in the reservoir, billions of dollars are invested in enhanced oil recovery researches to get the maximum amount of recovery with the lowest possible cost from the existing fields before moving to the remote areas.
AIMS AND OBJECTIVES
The aim of the study is to examine comparative analysis of miscible and immiscible gas flooding in enhancing oil recovery. Other specific objectives include:
1. to determine the relationship between miscible and miscible gas flooding and oil recovery.
2. to examine the effect of miscible and miscible gas flooding in enhancing oil recovery.
3. to carry out a comparative analysis of miscible and immiscible gas flooding in enhancing oil recovery.
4. to investigate the challenges towards enhancing oil recovery.
5. to proffer solution to the challenges towards enhancing oil recovery.
RESEARCH QUESTION
1. What is the relationship between miscible and miscible gas flooding and oil recovery?
2. What is the effect of miscible and miscible gas flooding in enhancing oil recovery?
3. What is the comparative analysis of miscible and immiscible gas flooding in enhancing oil recovery?
4. What are the challenges towards enhancing oil recovery?
5. What is the solution to the challenges towards enhancing oil recovery?
SIGNIFICANCE OF STUDY
The findings of this study will be of immense benefit to the oil sector like the NNPC and others on the effect miscible and immiscible gas flooding has in enhancing oil recovery.
Form this study, those in the oil sector will be exposed to a new knowledge in oil discovery.
Intending oil ventures can use this study to widen their knowledge on ways they can discover oil and as well as enhance their knowledge of oil discovery as the case may be.
Finally, the study can be used for further study and investigation in related topic or research.
SCOPE OF STUDY
The scope of study will cover comparative analysis of miscible and immiscible gas flooding in enhancing oil recovery.
LIMITATION OF STUDY
1. Financial constraint- Insufficient fund tends to impede the efficiency of the researcher in sourcing for the relevant materials, literature or information and in the process of data collection (internet).
2. Time constraint- The researcher will simultaneously engage in this study with other academic work. This consequently will cut down on the time devoted for the research work.
Do You Have New or Fresh Topic? Send Us Your Topic
DEFINITION OF TERMS
Comparative Analysis: A comparative analysis is an essay in which two things are compared and contrasted. You may have done a “compare and contrast”
Miscible: Miscible liquids are those liquids which upon mixing form one phase and no distinct layers are visible. for example vinegar solution.
Immiscible: Immiscible Liquids are said to be immiscible if they’re completely insoluble in each other. Such a system actually consists of 2 phases, though it is usually referred to as a mixture. Examples include benzene and water, kerosene and water, etc.
Gas flooding: Gas flooding is a form of oil recovery wherein the energy required moving the oil from the reservoir rock into a producing well is supplied from the surface by means of water injection and the induced pressure from the presence of additional gas.
Enhance: intensify, increase, or further improve the quality, value, or extent of.
Enhanced Oil recovery:
Enhanced oil recovery (abbreviated EOR), also called tertiary recovery, is the extraction of crude oil from an oil field that cannot be extracted otherwise. EOR can extract 30% to 60% or more of a reservoir’s oil, compared to 20% to 40% using primary and secondary recovery. According to the US Department of Energy, there are three primary techniques for EOR: thermal, gas injection, and chemical injection.
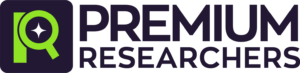
COMPARATIVE ANALYSIS OF MISCIBLE AND IMMISCIBLE GAS FLOODING IN ENHANCING OIL RECOVERY
INSTRUCTIONS AFTER PAYMENT
- 1.Your Full name
- 2. Your Active Email Address
- 3. Your Phone Number
- 4. Amount Paid
- 5. Project Topic
- 6. Location you made payment from